Editorial Disclaimer
The views expressed in articles published on FIRES do not necessarily reflect those of IES or represent endorsement by the IES.
By Timothy Brown, University of Manchester
The need for guidance
Across human history, we have used “artificial” lighting to allow us to interact with our environment and perform tasks under conditions where the scarcity of daylight would otherwise prove an impediment. Over the years we have developed lighting technologies to better approximate the perceptual qualities of daylight (i.e., white light that enables us to appropriately distinguish colours) and produced regulations for the built environment to specify precisely how much light should illuminate the local environment to support the visual demands of the task at hand. To provide a unified framework for comparing different types of lighting, a cornerstone of such regulations are photometric measures (e.g., lux) based upon an empirically defined function for weighting light energy at different wavelengths according its perceived brightness [V(λ)].1
It has been clear for many years, however, that light can also exert important influences on our brains and bodies that are not consciously perceptible, such as regulating our internal circadian clock system, hormone secretion, alertness and sleep.2-4 These “non-visual” responses to light are evolutionary ancient adaptations to our rotating planet whose purpose is to tune brain and body function across the day and night as appropriate for the diurnal changes in the natural world. As our ability to create light on demand has freed us from a reliance on the sun for activities requiring vision, it has dramatically changed our daily patterns of light exposure, resulting in far greater light exposure during the evening and night and substantially less during the daytime (due to our increasing reliance on indoor electric lighting and reduced exposure to daylight). This has raised concerns that our modern patterns of light exposure disrupt the normal daily biological control mechanisms and negatively impact health, well-being and performance.5-7 Until recently, however, we have lacked explicit direct guidance on precisely how much light is enough to appropriately recreate “biological daytime” and what amount is too much to appropriately signal “biological night.”
Historical hurdles
By contrast to our existing regulations pertaining to vision, a particular challenge in defining guidelines relating to biologically appropriate light exposure has been the question of how to reliably quantify the propensity of light to engage the appropriate responses. Hence, around the turn of the century we learnt that the biological origins of these subconscious effects of light on our brains and bodies were at least partially separable from those underlying visual perception; blind individuals lacking conscious light perception could retain non-visual responses to light, and the ability of light of different wavelengths to trigger such effects in sighted individuals (e.g., supressing secretion of the hormone melatonin) was not accounted for by existing photometric measures.8-11 Around the same time, human and animal research identified the biological mechanism responsible with the discovery of a new class of light-sensing cell in the eye, the intrinsically photosensitive retinal ganglion cells (ipRGC).12-14 In particular, the ipRGCs were found to possess their own specialised light sensing protein – melanopsin – whose sensitivity to light is substantially biased towards shorter wavelengths15 than is the V(λ) function, which reflects the properties of the cone cells we rely on for photopic vision and which underlie photometric measures of brightness (Figure 1).
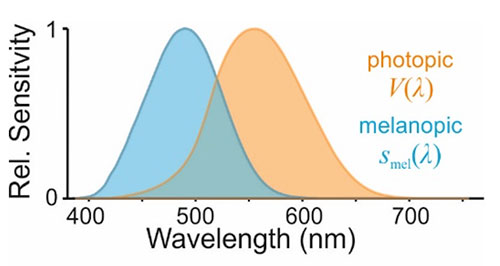
While the discovery of melanopsin helped explain the inability of photometry to account for the non-visual effects of light, it also quickly became apparent that the regulation of these responses could be quite complex. For example, in addition to their inherent melanopsin-dependent light sensing, the ipRGCs can integrate signals from the rod and cone cells in the retina and convey these to the brain.16 Thus, in principle, any of the five photoreceptor types in humans (ipRGCs, rods and the three cone types) could influence human non-visual responses to light. The relative contributions of these different kinds of photoreceptive signals have thus been an important research question, with accumulating data over the past 20 years suggesting this could vary according to various features of the light exposure and perhaps also between different types of non-visual responses (Figure 2).
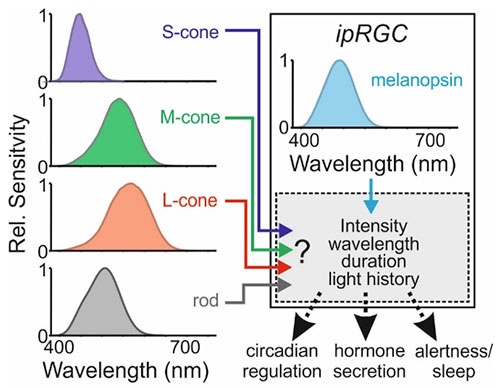
Pathway to defining guidance
As an initial response to the need to define an appropriate metric to quantify the propensity of light to drive non-visual responses, an expert working group convened (Manchester, 2013) with a view to establishing a scientific consensus as to the best path forward.17 Given the then uncertainty as to how signals from the different photoreceptor classes were combined to define the effective spectral sensitivity of non-visual responses, the interim solution proposed was a set of five (α-opic) spectral weighting functions corresponding to the five human photoreceptor classes that might contribute (Figure 2). That approach was then subsequently adopted and extended in the recent international standard for metrology of ipRGC-mediated responses (CIE S 026),18 with modifications to make the resulting metrics fully SI compliant. For example, the new system provides a means by which the photoreceptor-weighted (i.e., α-opic) irradiances of any light source can be expressed in terms of the photopic illuminance from 6500-K daylight required to produce the equivalent α-opic irradiance (equivalent daylight illuminance, EDI; units: lux).
As originally envisioned, this new measurement framework facilitated a range of retrospective evaluations of historical data examining the spectral sensitivity of circadian, endocrine and alerting responses to light,19-21 as well as key, hypothesis-led investigations22-24 that, collectively, removed much of the original uncertainty as to the contributions of different photoreceptors. Given these advances, we convened a second international expert working group (Manchester, 2019), with the goal of establishing quantitative recommendations for biologically appropriate light exposure, grounded upon the new measurement standard.25 Based on the new insights available, which clearly support the view that melanopsin is the primary determinant of non-visual spectral sensitivity, the consensus view of the participants that emerged from that workshop was that melanopic EDI is the best metric upon which to base such recommendations. Hence, under the most practically relevant situations (i.e., extended exposure to “white” light), the available research data indicates that any contributions from the additional photoreceptor classes is not sufficiently pronounced so as to render this an unreliable predictor of the magnitude of the expected biological responses. Moreover, the consistency in the range of melanopic EDI at which important effects on the circadian system, hormone secretion and alertness are observed provided a straightforward basis upon which to define quantitative threshold recommendations for biologically appropriate light exposure (Figure 3).
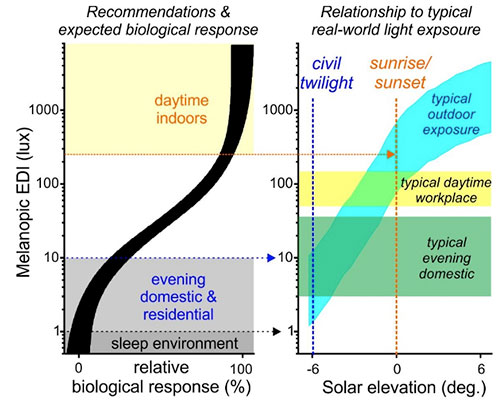
Accordingly, while it has long been clear that in order to support daytime alertness and activity, restful sleep and overall healthy physiology, our days should be “bright” and our nights “dark,” there is now scientifically agreed, quantitative guidance as to what these should entail. Hence, for the daytime, the recommended minimum melanopic EDI of 250 lux (vertically at eye level) is expected to appropriately maximise the biological signal of “day” to brain systems regulating circadian rhythms and arousal. By contrast, for the sleep environment, where even low levels of light exposure can exert disruptive effects on circadian rhythms, sleep and physiology, keeping light exposure below a melanopic EDI of 1 lux should provide an appropriate signal of biological “night” to minimise such issues. In between these two extremes, there is of course a period in the evening where we require light to see but in which that light can potentially exert disruptive effects on circadian rhythms and subsequent sleep. Accordingly, the recommended maximum melanopic EDI of 10 lux during the evening (beginning 3 hours before bedtime) is intended to mitigate, so far as possible, this issue while still providing sufficient light for common evening activities.
Practical applicability
The intent behind the new recommendations is to provide a straightforward framework that describes a biologically appropriate, healthy, daily pattern of light exposure and, in turn, to inform lighting design and practice that aim to create this in indoor settings. Any such endeavour must of course also balance that goal with a range of other factors that already influence lighting choices, from existing regulations specifying appropriate illuminance levels to considerations around energy efficiency, visual comfort and aesthetic factors.26 It is, therefore, important to consider the extent to which these recommendations are applicable and achievable in real-world applications as part of “integrative” lighting practice.
First, whom are the recommendations intended to apply to? Given that the overwhelming majority of the scientific data upon which they are based derives from healthy working-age adults, they are principally formulated with that group in mind.25 Importantly, however, while there is evidence that some groups (e.g., children and older adults) may be more or less sensitive to light than the target demographic, there is a strong expectation that such individuals would also benefit from the specified recommendations (insofar as these entail increased daytime and reduced evening and/or nighttime light exposure). A more challenging area, and one where the recommendations are not intended to apply, is for those individuals who do not have regular daytime schedules (e.g., shift workers), where potentially beneficial effects of light (e.g., promoting alertness) may be accompanied by detrimental effects (e.g., on the circadian system and sleep). Outside of that group, however, the recommendations should be broadly applicable and beneficial, and therefore relevant across a range of environments from offices, educational and healthcare establishments to domestic and residential settings.
Second, how achievable are these recommendations? In the context of evening and nighttime lighting in domestic settings, they are actually already in line with what many individuals currently experience.27 Moreover, in the remaining cases, there will often be straightforward approaches to appropriately reduce light exposure. For example, switching from currently available cool white (6500 K) to warm white (3000 K) LED lighting would, in its own right, reduce melanopic EDI by more than 50%.28 Alongside other strategies to minimise unnecessary light exposure (e.g., use of appropriate task lighting, blackout blinds in the bedroom), the target levels for evening and nighttime should be readily achievable in most domestic settings. On the other hand, throughout much of the day, the majority of individuals will currently experience light levels somewhat (approximately 30% to 60%) below the recommended minimum melanopic EDI of 250 lux.25 Fortunately, here there are also various approaches that could remedy this. For example, commonly used office fluorescent and LED lighting (4000 K) provides a relatively low ratio of melanopic EDI to photopic illuminance (termed –melanopic daylight efficacy ratio; melanopic DER; see Figure 4). Increasing this, either via newer high melanopic-content bulbs and/or increased access to daylight, constitutes one clear route to increase melanopic light exposure without increasing overall illuminance. Alongside other strategies to increase eye-level melanopic light exposure (e.g., considering the placement, angular dispersion and size of accessible luminous surfaces and optimising the reflectance of furnishings and finishes), achieving the recommended daytime target need not require significant compromises on energy efficiency or visual comfort.
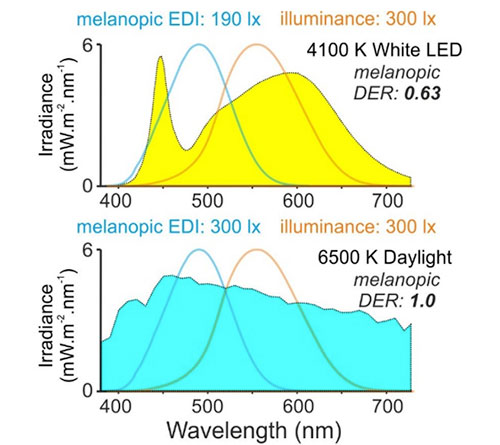
In sum, while optimising lighting to meet our biological needs will require careful consideration and design, it is in no way an insurmountable challenge or one that should require major compromises in other areas. With the availability of tools to calculate and model melanopic EDI29-31 to aid planning, and ongoing advances in solid state lighting, the new recommendations25 provide an important framework to inform the design and use of lighting such that it not only supports our visual requirements, but also health, well-being and performance.
References
1 CIE 018: 2019. THE BASIS OF PHYSICAL PHOTOMETRY, 3RD EDITION. (CIE Central Beareau, Vienna, 2019).
2 Paul S, Brown, T. (2019). Direct effects of the light environment on daily neuroendocrine control. J Endocrinol. 243, R1-R18
3 Souman JL, Tinga AM, Te Pas SF, van Ee R, Vlaskamp BNS. (2018). Acute alerting effects of light: A systematic literature review. Behavioural brain research 337, 228-239.
4 Santhi N, Ball DM. (2020). Applications in sleep: How light affects sleep. Prog Brain Res 253, 17-24.
5 Lunn RM, Blask DE, Coogan AN, Figueiro MG, Gorman MR, et al. (2017). Health consequences of electric lighting practices in the modern world: A report on the National Toxicology Program’s workshop on shift work at night, artificial light at night, and circadian disruption. Sci Total Environ 607-608, 1073-1084.
6 Mason IC, Boubekri M, Figueiro MG, Hasler BP, Hattar S, et al. (2018). Circadian Health and Light: A Report on the National Heart, Lung, and Blood Institute’s Workshop. J Biol Rhythms 33, 451-457.
7 Boyce PR. (2021). Light, lighting and human health. Lighting Res. Technol. EPUB.
8 Czeisler CA, Shanahan TL, Klerman EB, Martens H, Brotman DJ, et al. (1995). Suppression of melatonin secretion in some blind patients by exposure to bright light. N Engl J Med 332, 6-11.
9 Brainard GC, Hanifin JP, Greeson JM, Byrne B, Glickman G, et al. (2001). Action spectrum for melatonin regulation in humans: evidence for a novel circadian photoreceptor. J Neurosci 21, 6405-6412.
10 Thapan K, Arendt J, Skene DJ. (2001). An action spectrum for melatonin suppression: evidence for a novel non-rod, non-cone photoreceptor system in humans. J Physiol 535, 261-267.
11 Klerman EB, Shanahan TL, Brotman DJ, Rimmer DW, Emens JS, et al. (2002). Photic resetting of the human circadian pacemaker in the absence of conscious vision. J Biol Rhythms 17, 548-555.
12 Provencio I, Rodriguez IR, Jiang G, Hayes WP, Moreira EF, et al. (2000). A novel human opsin in the inner retina. J Neurosci 20, 600-605.
13 Berson DM, Dunn FA, Takao, M. (2002). Phototransduction by retinal ganglion cells that set the circadian clock. Science 295, 1070-1073.
14 Hattar S, Liao HW, Takao M, Berson DM, Yau KW. (2002). Melanopsin-containing retinal ganglion cells: architecture, projections, and intrinsic photosensitivity. Science 295, 1065-1070.
15 Bailes HJ, Lucas RJ. (2013). Human melanopsin forms a pigment maximally sensitive to blue light (lambdamax approximately 479 nm) supporting activation of G(q/11) and G(i/o) signalling cascades. Proc. Roy Soc Biol Sci 280, 20122987.
16 Do MTH. (2019). Melanopsin and the Intrinsically Photosensitive Retinal Ganglion Cells: Biophysics to Behavior. Neuron 104, 205-226.
17 Lucas RJ, Peirson SN, Berson DM, Brown TM, Cooper HM, et al. (2014). Measuring and using light in the melanopsin age. Trends in neurosciences 37, 1-9.
18 CIE S 026/E:2018. CIE System for Metrology of Optical Radiation for ipRGC‐Influenced Responses to Light. (CIE Central Bureau, Vienna, 2018).
19 Nowozin C, Wahnschaffe A, Rodenbeck A, de Zeeuw J, Hadel S, et al. (2017). Applying Melanopic Lux to Measure Biological Light Effects on Melatonin Suppression and Subjective Sleepiness. Curr Alzheimer Res 14, 1042-1052.
20 Prayag AS, Najjar RP, Gronfier C. (2019). Melatonin suppression is exquisitely sensitive to light and primarily driven by melanopsin in humans. J Pineal Res 66, e12562.
21 Brown TM. (2020). Melanopic illuminance defines the magnitude of human circadian light responses under a wide range of conditions. J Pineal Res, e12655.
22 Allen AE, Hazelhoff EM, Martial FP, Cajochen C, Lucas RJ. (2018). Exploiting metamerism to regulate the impact of a visual display on alertness and melatonin suppression independent of visual appearance. Sleep 41.
23 Souman JL, Borra T, de Goijer I, Schlangen LJM, Vlaskamp BNS, et al. (2018). Spectral Tuning of White Light Allows for Strong Reduction in Melatonin Suppression without Changing Illumination Level or Color Temperature. J Biol Rhythms 33, 420-431.
24 de Zeeuw J, Papakonstantinou A, Nowozin C, Stotz S, Zaleska M, et al. (2019). Living in Biological Darkness: Objective Sleepiness and the Pupillary Light Responses Are Affected by Different Metameric Lighting Conditions during Daytime. J Biol Rhythms 34, 410-431.
25 Brown TM, Brainard GC, Cajochen C, Czeisler CA, Hanifin JP, et al. (2022). Recommendations for daytime, evening, and nighttime indoor light exposure to best support physiology, sleep, and wakefulness in healthy adults. PLoS biology 20, e3001571.
26 Houser KW, Boyce PR, Zeitzer JM, Herf M. (2021). Human-centric lighting: Myth, magic or metaphor? Lighting Res. Technol. 53, 97-118.
27 Cain SW, McGlashan EM, Vidafar P, Mustafovska J, Curran SPN, et al. (2020). Evening home lighting adversely impacts the circadian system and sleep. Sci Rep 10, 19110.
28 Schlangen LJM, Price LLA. (2021). The Lighting Environment, Its Metrology, and Non-visual Responses. Front Neurol 12, 624861.
29 CIE S 026 a-oic toolbox. (CIE Central Bureau, Vienna, 2020).
30 Spitschan M, Mead J, Roos C, Lowis C, Griffiths B, et al. (2021). luox: novel open-access and open-source web platform for calculating and sharing physiologically relevant quantities for light and lighting. Wellcome Open Res 6, 69.
31 Safranek S, Collier JM, Wilkerson A, Davis RG. (2020). Energy impact of human health and wellness lighting recommendations for office and classroom applications. Energy & Buildings, 110365.