Editorial Disclaimer
The views expressed in articles published on FIRES do not necessarily reflect those of IES or represent endorsement by the IES.
By Ian Ashdown, KC Fletcher, and Emil Radkov
For over a century, lighting designers have modeled luminaires as point light sources. This made perfect sense for a half-century of incandescent lamps. However, when linear fluorescent lamps were introduced in the late 1940s, it became clear that the point source model had its limits. To accurately calculate the illuminance distribution of a surface, its distance to the lamp had to be at least five times the lamp length. (This is the basis of the “five-times rule” for far-field photometry.)
The luminous area of the luminaire is typically modeled as a one- or two-dimensional array of point sources with prorated intensity values for radiosity methods. Another approach uses randomly selected points on the luminous area surface as the origin of direct light rays for ray tracing methods. In a third approach, the luminous area is modeled directly using proprietary methods.
Today, we have need of more accuracy in our lighting designs. Looking beyond artful manipulations by lighting design software, we need to improve how we measure the optical characteristics of luminaires.
Industry needs
In 2000, the IES published IESNA LM-70-00, Approved Guide to Near-Field Photometry. This document was intended to improve the accuracy of illuminance [lux] modeling in near-field applications such as task lighting, wall washing, and indirect lighting. Even at that time, many lighting designers knew that using the data from far-field characterizations of a luminaire would often yield inaccurate results when applied to the near field. Unfortunately, LM-70 was withdrawn due to limited practice in the industry.
However, in the late 2010s and early 2020s, multiple industries parallel to the general illumination industry began presenting the need to more accurately model in near-field applications. This led to the effort that resulted in the publication this year of ANSI/IES LM-91-22, Approved Method: Application Distance Photometry.
One such industry was the horticultural lighting industry, where, instead of calculating illuminance, end users were interested in photon flux density (or photon irradiance) [μmol/s·m2]. Optimizing the uniformity under a luminaire was crucial for uniform crop production, and in many applications, the lights were being installed very close to the canopies.
Another industry that presented the need was the UV-C germicidal industry. Given that awareness had been increasing regarding healthcare-acquired infections (HAIs), and given the immergence of the COVID-19 pandemic, the UV-C industry needed accurate modeling of irradiance [W/m2]. The goal of industry was to optimize the dose [mJ/cm2] on a surface, which often required that the application plane was as near as possible to the source.
These two industries alone were enough to motivate lighting metrology labs to want to standardize testing methods for accurately characterizing light sources in the near field, and standardize the format of the results such that they could easily be adopted by these industries. The publication of ANSI/IES LM-91-22 will not only benefit these two industries; it will also replenish the needs that LM-70 was intended to address.
Shortcomings of far-field point-source data
Goniometric intensity data for luminaires are usually collected under far-field conditions, i.e., from a distance exceeding by at least five times the largest luminous dimension of the luminaire. From those data, a far-field photometric file can be then constructed by projecting them from a point of origin, which is set conventionally at the photometric center of the luminaire. There are several formats available for such files (for example, LM-631 and TM-332 in the U.S., and EULUMDAT in Europe), which list the intensity data along with some basic geometric information for the luminaire and its luminous surface. The latter information becomes important whenever one needs to use the photometric file under near-field conditions, i.e., at working distances comparable to the luminaire size. In such cases, a point representation of the luminaire could lead to very inaccurate results with far-field intensity data.3,4
Many popular lighting application modeling software packages employ techniques for more accurately predicting nearfield illuminance from far-field data. It should be noted, however, that these techniques make certain assumptions about the luminaire that may highly affect the accuracy of the calculations.3,4 These techniques are also highly dependent on the dimensions of the luminaire’s luminous opening as embedded in the far-field data file.
The above approach works well if the light-emitting surface of the luminaire has a good match within the rather limited set of basic geometric shapes available in the photometric file standards, such as LM-63-19, but can introduce significant errors when no such match exists. In certain cases, it may be possible to break down the luminaire geometrically into subunits of simpler shape (for example, a set of rectangular luminous surfaces for a luminaire consisting of several linear subunits). However, this increases the complexity of the lighting model and may require additional manipulation of the far-field luminaire data, such as intensity proration to any particular subunit under consideration.
Furthermore, the modeling software itself could be using an approximation of the luminous shape encoded in the photometric file—for example, by representing a cylinder (or even a sphere) as a prism—thereby introducing additional errors in the near-field lighting model.
All of the preceding problems and complications are eliminated by collecting near-field intensity data and using them to construct a near-field photometric file by following the new ANSI/IES standard LM-91-22.5 This standard is not limited to luminous intensity; it can also be used for radiant or photon intensity equally well.
Data Acquisition and representation
According to ANSI/IES LM-91-22, near-field data are collected on a measurement plane, using either a rectangular grid (for the creation of simple illuminance, irradiance, or photon flux density maps) or a grid in cylindrical coordinates (for the construction of IES files to be used in modeling). In the latter case, the raw data are acquired on the measurement plane at a distance z from the luminaire with a cosine-corrected detector oriented along the z-axis, and are correlated to an angular measurement grid by using the following formula, taking into account the direct distance r from the optical center of the luminaire and the vertical angle q, at which the detector is seen from the optical center:
r = z ∙ tan θ
Figure 1 illustrates a typical measurement grid, assuming two perpendicular mirror planes of symmetry.
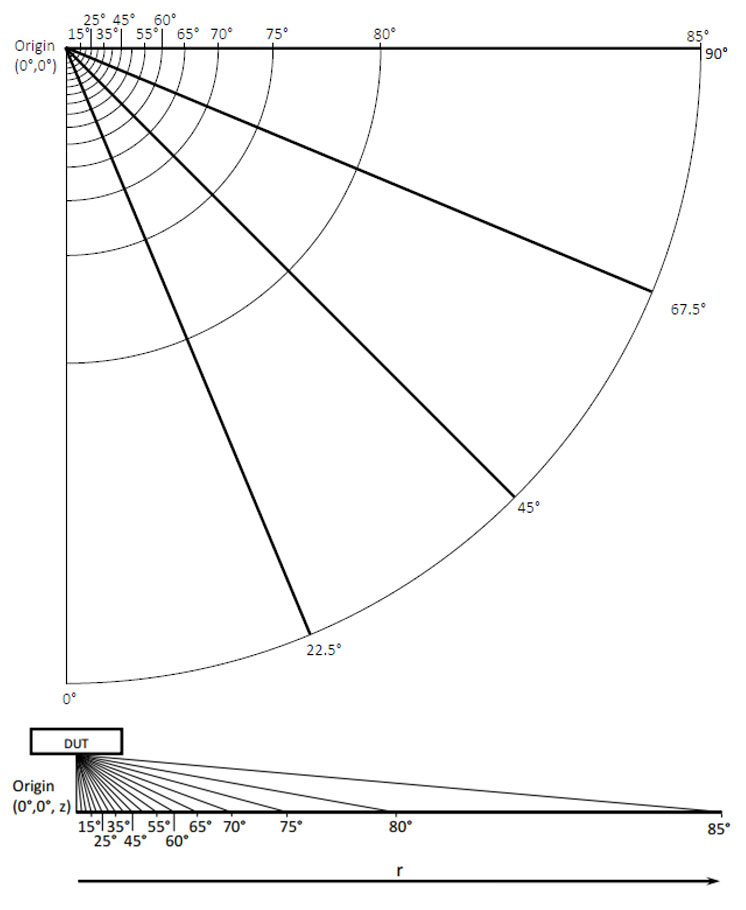
From there, the raw illuminance, irradiance, or photon flux density data are converted to the corresponding intensity units and then corrected, such that the partial luminous (or radiant or photon) flux calculated in the near field matches the flux calculated in the far field over the same angular range in the diagram. This follows from the basic physical expectation that the total flux emitted from a given luminaire must be the same regardless of the measurement method used.
Finally, a near-field photometric file is compiled by following additional rules allowing the use of far-field intensity data beyond certain vertical angles, where collection in the near field becomes impossible or impractical and where a far-field approximation is applied instead. Examples of such files are given at the end of ANSI/IES LM-91-22, using the IES formats standardized in ANSI/IES LM-63-19 and ANSI/IES TM-33-18.
The resulting near-field photometric file can be used directly with lighting design software under the following key conditions: 1) the distance from the luminaire to the calculation plane matches the z-distance to the measurement plane used for the near-field photometric file; and 2) the luminaire size is represented as a point (or its smallest available approximation by any software not supporting point sources). The need for the first condition is obvious. The second condition arises from the mathematical procedure used to construct that particular file (projection from a single point of origin, set at the optical center of the luminaire). Important: Using the actual luminous dimensions of the luminaire in a lighting calculation can be expected to give incorrect results with such a file.
It should be noted that although file formats such as LM-63, TM-33, and EULUMDAT are compatible with near-field photometric files generated per ANSI/IES LM-91-22 (with the TM-33 format natively supporting that standard), no modeling software currently in use possesses a built-in check for the above two conditions with near-field files. It is therefore the responsibility of the end user to ensure that the requisite conditions are met in any given model, until lighting design software explicitly supporting near-field intensity data becomes available.
Measurement challenges
A major and specific challenge in near-field measurements (besides the need for a testing setup capable of carrying them out on the measurement plane in the first place) is procuring a cosine-corrected detector with small enough deviations from the ideal cosine response. Extensive modeling by the LM-91 working group (summarized in Annex A of ANSI/IES LM-91-22) has demonstrated the critical impact of such deviations on the accuracy of the resulting data. That impact becomes particularly pronounced at shorter application distances where the detector views the luminaire from higher angles, at which deviations from the ideal cosine response tend to increase. Accordingly, a special test protocol is provided in ANSI/IES LM-91-22 to determine the highest vertical angle (and from there, the minimal application distance) to which near-field measurements may be carried out with any given detector. The shortest application distance implied by that protocol is just under 15 cm (6 in.) for a detector complying with the specified upper and lower cosine response deviation limits out to 85o.
Another measurement challenge (also present in far-field measurements) is the need for increased angular scanning resolution when the beam angle of the device under test (DUT) decreases below 120 degrees. Based again on extensive modeling of DUTs with various beam angles, specific minimal scan resolutions are required in ANSI/IES LM-91-22 according to beam angle, as determined by far-far-field goniometry. Although not expressly forbidden in ANSI/IES LM-91-22, measurements of DUTs with beam angles less than 58° are not supported by that standard due to the expected sharp increase in necessary angular resolution. The same applies to measurement planes closer to the DUT than 15 cm, regardless of beam angle.
Processing of near-field data for IES file generation starts with the straightforward step of transforming the raw measurements into intensity units based on a formula given in ANSI/IES LM-91-22, followed by a correction for the partial flux based on numerical integration, also using a given formula. These steps can be done in a spreadsheet or by other software customized for the testing laboratory. A somewhat more unusual step is the merging with far-field data, which may also be necessary at and beyond a certain vertical angle, as described in the standard. This allows for additional modeling capability of the resulting IES file in the far field, on and away from the application plane when needed. Finally, certain specific formatting and language are required for the near-field IES files, as shown in Section 9.4 and by the examples provided in Annex B of ANSI/IES LM-91-22.
Reporting requirements
Some of the reporting requirements in ANSI/IES LM-91-22 are driven by the applicable technology-specific normative document(s): ANSI/IES LM-79-19, ANSI/IES LM-41-20, ANSI/IES LM-46-20, and ANSI/ASABE S642. There are also additional details required specifically in ANSI/IES LM-91-22, such as administrative information (testing agency identification, testing, and report date), DUT description (manufacturer name and model number), test conditions, test equipment (including wavelength range), and measured results (electrical input parameters, application distance(s), coordinate system used, measurement points, and measured optical data, as applicable). Any additional information required by the test requestor or regulatory body may also be added into the test report.
References
- Illuminating Engineering Society. ANSI/IES LM-63-19, Approved Method: IES Standard File Format for the Electronic Transfer of Photometric Data and Related Information. New York: Illuminating Engineering Society; 2019.
- Illuminating Engineering Society. ANSI/IES TM-33-18, Standard Format for the Electronic Transfer of Luminaire Optical Data. New York: Illuminating Engineering Society; 2018.
- Murdoch J. Inverse square law approximation of illuminance. J Illuminating Engineering Society. 1981;10(2):96-106.
- Saraiji RMN, Mistrick RG. Calculation methods, error tendencies, and guidelines for finite element flux transfer. J Illuminating Engineering Society. 1992;21(1):92-102.
- Illuminating Engineering Society. ANSI/IES LM-92-22, Approved Method: Optical and Electrical Measurement of Ultraviolet LEDs. New York: Illuminating Engineering Society; 2022.